Outside, clouds glide across the horizon. A man on the street below seems in a hurry. Two cars and a lorry drive past him. And a sparrow lands on the windowsill. As you get up to see it closer, a pencil rolls off the end of the table and falls to the floor. None of this may seem particularly eventful to you but it does take a very intricate network of cells to process the surrounding information so that you can see it all happening. Most of us take eyesight for granted. Most of us take the perception of movement for granted too. Without all the specialized cells that make up an eye, it would be a very difficult world to move around in, but also to apprehend. What is more, not only is the information of our surroundings relayed and processed in a fraction of a second, but we can effortlessly distinguish between what is moving, and what is not. Even if we are, ourselves, moving. Recently, scientists discovered that the ability to see objects in motion does not use the same system as the one we use to perceive a tree standing still in a park for instance. In fact, the system it uses seems to slow the process of perception down a tad, and is made possible thanks to a protein known as sidekick 2.
Understanding how the outside world is brought to us, so to speak, by our inside world has been the source of a few theories over the centuries. The Ancient Greeks were the first to provide a rather crude explanation of what eyesight could be. There were two schools. The first believed that the sight of an object is made possible by way of rays that emanate from our eyes and intercept it. The second believed that it was, on the contrary, something that emanated from the object itself which entered our eyes. In the 18th century, scientists such as the physicist Isaac Newton and the philosopher John Locke blended the two theories and suggested that visual perception was in fact the result of rays that emanated from objects and entered the mind through the eye - thus shifting the paradigm a little and making the mind the seat of visual perception. It is the German physician Hermann Helmholtz (1821-1895) who is credited with the more modern understanding of visual perception. And 20th century technology, coupled with huge leaps in the field of molecular biology, has brought us to our current - albeit incomplete - understanding of visual perception, which is one of extreme complexity.
What we now know is that an eye is an intricate mesh of all sorts of sensory cells whose job is to relay light in all its wavelengths from the outside world - via the retina - to our brain, which in turn processes the information and feeds it back to tell us what "we see". Relaying outside signals to the inside is done via synapses. Light enters our eyes where it is converted into electrical signals by photoreceptor cells in the retina. These electrical signals are then relayed by interneurons - via their synapses - to the retinal ganglion cells, or RGCs. RGCs then relay the messages deeper into our brain where they are processed. All this happens within a fraction of a second. In the mammalian retina there are about 70 different types of neurons that form specific synapses on about 30 types of RGCs. And all these neurons, synapses and ganglion cells are intertwined to form a most complex network, much of which has still to be understood.
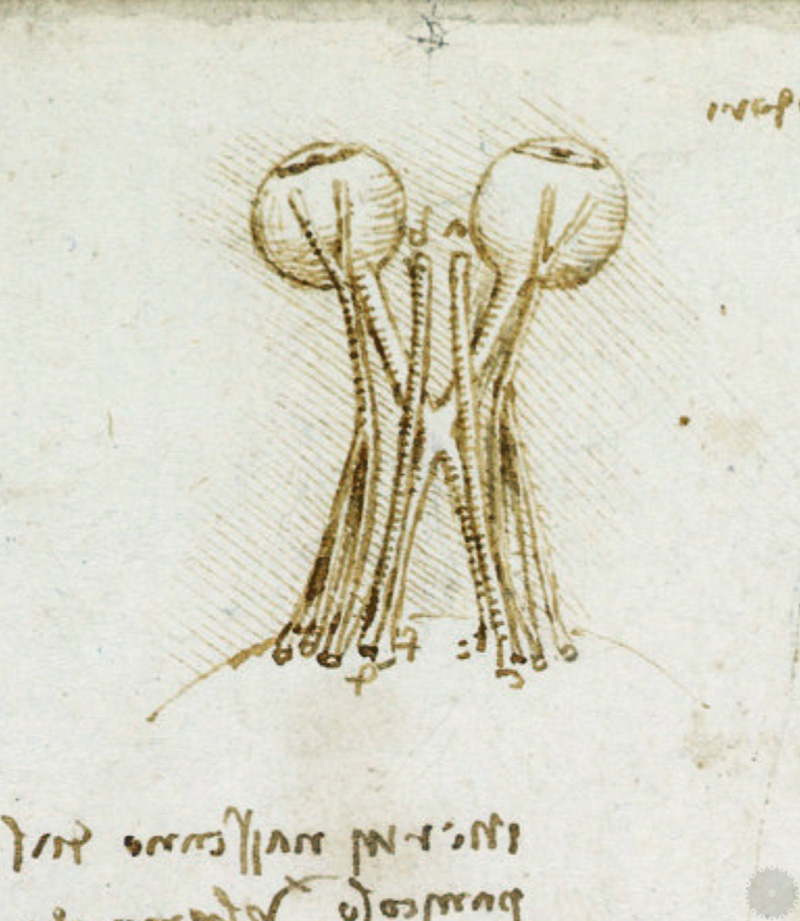
However, recently, scientists discovered that being able to discern something that is moving -even when we ourselves are moving - takes a slightly different pathway. What is more, it seems to take a pathway that delays the signal - which seems an odd option. Why delay a signal that is so vital to a living being? The RGCs that are able to sense motion have been called object motion sensors. These particular RGCs are slower to react to light than the more "routine" RGCs. It is thought that the time lapse created is necessary for information resulting from our peripheral vision and that of our central field of vision to arrive at the same time and position in our brain, where both are taken into account simultaneously and processed as a coherent whole. In this way, the brain is able to distinguish objects within our field of vision that are moving at different speeds.
But what is it that creates this essential delay for discerning motion? Scientists discovered that object motion sensors communicate - i.e. form synapses - with extra sensor cells known as VG3 amacrine cells. Whereas perceiving an object that is immobile demands a relay of information between a photoreceptor cell, an interneuron and an RGC, an object in motion seems to demand an extra relay, i.e. a photoreceptor cell, an interneuron, an amacrine cell and finally an RGC which sends the signal deeper into the brain. It is not difficult to grasp that an extra relay demands extra time. What is more, scientists discovered that both the amacrine cells and the motion sensor cells express a protein known as sidekick 2 exactly where the two cells come into contact. Sidekick 2 is an immunoglobulin superfamily recognition molecule and acts as an adhesion molecule between the two types of cells by first helping them to find each other and then bind to each other, thus making the connection highly selective. The synapses involved in such a connection are directly involved in relaying information between the VG3 amacrine cells and their specific contacts, W3B-RGC cells. In the absence of sidekick 2, connections between the two cells fail.
Understanding the intricacy of the molecular pathways that lead to visual perception will serve to find ways of improving a person's vision which is poor, and perhaps even blindness. Getting the connections right between the various neuronal cells involved in eyesight is essential. Seeing colour, discerning an object or perceiving moving objects depend on it. But, like the neuronal network that is the seat of our brain, the mesh of neurons that is at the heart of visual perception is proving to be extremely complex. In the retina, all the different types of neurons intermingle as they develop while connecting up to one another properly. All these processes demand many more years of research to understand in detail. So far, the research has been carried out on mice but scientists hope to be able to study the same phenomena in the human eye, with a view to imagining innovative treatments for vision problems.